PARE ARTICLE - EMERGING AVIATION TECHNOLOGIES
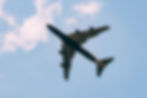
To support the 23 Flightpath 2050 goals established by the Advisory Council for Aeronautics Research and Innovation in Europe (ACARE), the PARE project defined 35 complementary PARE objectives. Objectives 29 to 36 are addressed in the 8th chapter of PARE's 2nd-year report, entitled “Emerging Aviation Technologies”, which concern new disrupting technologies that are reshaping the aviation sector.
ELECTRIC PROPULSION AND ELECTRIFIED AEROPLANE COMPONENTS
Although the automobile sector may lead the electrification of transport vehicles, the specific needs of aeronautics and fast technological evolution, in particular advances in electric propulsion (EP) and systems, will have increasing importance from drones to aeroplanes, including convertibles (those capable of vertical take-off and landing (VTOL)) taxis. Moreover, many components/systems of conventional aircraft (e.g. actuation, de-icing, and air-conditioning), which use mechanical, hydraulic and pneumatic power sources have been replaced throughout the years by electrical ones due to lack of reliability and high maintenance costs. Considering this, by 2050, the EU must be kept at the forefront of progress in the electrification of aircraft, including components.
Key Findings:
EP uses electrochemical power sources (ECPS) such as batteries or fuel (often hydrogen) cells (FC). Currently, it is more effective for small vehicles, with short or medium ranges with a low weight such as drones transporting measuring sensors. Nevertheless, strongest developments of EP also take place at VTOL taxis, normally with a range between 50 and 100 kilometres (km) and partially larger. Under development are also regional and business aircraft with targets around 500 km;The flight range of all-electric aircraft depends on the specific energy (Watt-hour per kilogram - Wh/kg) of the ECPS, besides engineering parameters (such as lift over drag ratio, battery mass fraction – mass battery / total aeroplane mass - and efficiency of powertrain). The today all-electric aircraft are limited to the low total weight, i.e. small passenger numbers and/or low payload as well as limited endurance and speed. Applications that fit reasonable these limitations are the ones stated in the first bullet point;Long-range transportation of a large number of passengers or heavy cargo will rely for some time to come on conventional propulsion that still has a considerable development potential on its own and as part of hybrid systems. Considering the latter, high flight ranges (≥ 1000 km) are possible with FC as ECPS, because their energy (and consequently the flight range) is determined mainly by the hydrogen, which has relatively low weight and contributes only insignificantly to the total aeroplane mass. Nevertheless, the costs of FC related to the power are higher as for batteries;Concerning battery-powered aircraft, solar-powered aircraft (SPA) that are solar generator/ECPS hybrids should eliminate the current disadvantage of all-electric aircraft. Furthermore, they offer the ultimate promise of environmentally clean operation for long periods up to a month or even years and the radiation increases with higher altitude. Nevertheless, a commercial introduction is only expected from 2035; “Fly by Wire” (FBW) systems include electrically actuated thrust reverser, hybrid electro-hydraulic actuation systems for wing and tail flight control surfaces, electrically powered environmental control (air-conditioning) system, electrically actuated brakes etc. The higher electrification leads to reduced weight, greater reliability, lower maintenance costs and increased efficiency and of course lower emissions.
Key Actions:
To achieve PARE objective 29, it is recommended that a thorough assessment is made followed by support measures on (a) emerging electric systems and propulsion technologies, (b) their potential to satisfy mission requirements and (c) the likely evolution of both.
ADDITIVE MANUFACTURING
Additive manufacturing allows the production of complex pieces with fewer parts and could replace spare part inventories with local production, as limitations in series production, choice of materials and quality finish are overcome. Although it is an industry-wide activity, aeronautics may be the leader in some areas and should keep up with progress in other areas. Taking this into account, by 2050, advances in additive manufacturing must be promoted and exploited.
Key Findings:
Additive manufacturing has several attractive features: (i) since it consists of adding layers, it does not waste material unlike processes such as milling that carve a part out of a larger block; and (ii) it allows the manufacture of large complex specimens that would otherwise have to be built-up of smaller parts with junctions. Thus, additive manufacturing is one of the contributors to more efficient production;It also has limitations: (i) it is a slow process more suited to laboratory use or limited production of prototypes than to large scale high-rate serial industry; and (ii) the surface smoothness is dependent on the number of layers that cannot be excessive, thereby requiring subsequent operations to ensure a better finish;If the limitations of additive manufacturing can be overcome there are promising prospects: (i) producing parts locally and as needed instead of transporting them from large stocks in a warehouse; and (ii) coupled with digital imaging and sensing producing replicas and replacements for damaged parts already out-of-stock or unavailable. These features will become more useful as the range of materials suitable for additive manufacturing and their physical properties widen.
Key Actions:
To accomplish PARE objective 30, it is recommended to consider the implications on prototyping, series production and spares supply of additive manufacturing regarding (a) usable materials, (b) quality standards and (c) life-cycle costs.
EFFICIENT PRODUCTION
The more complex world of aeronautics compared with other sectors, e.g. the automotive sector that has taken the lead on efficient production, provides opportunities for cross-fertilization in new technologies and their efficient utilization and management. The next stage in the evolution towards efficient production is known as “Industry 4.0” and aeronautics can benefit from 4.0 technologies, especially by integrating the full life-cycle design-production-operation-maintenance. Considering this, by 2050, the experience from other sectors should be incorporated to achieve more efficient and economical production in aeronautics.
Key Findings:
Some of the key concepts of efficient production read across production volume, value and rate and include: (i) just-in-time delivery avoiding large and costly static inventories; (ii) work planning that maximizes human motivation and efficiency; and (iii) the right mix of automation and human intervention; There are counter-factors that favor aeronautics regarding efficient production: (i) higher added value justifies bigger investments in quality and efficiency; and (ii) large order backlogs if they are achieved give a measure of long-term stability; The term Industry 4.0 refers to the combination of several major innovations in digital technology, all coming to maturity right now, all poised to transform the energy and manufacturing sectors. The following nine technology trends form the building blocks of Industry 4.0: (1) big data and analytics; (2) advanced robotics and artificial intelligence; (3) simulations; (4) horizontal and vertical system integration; (5) the industrial Internet of Things (IoT); (6) cybersecurity; (7) cloud computing; (8) additive manufacturing; and (9) augmented reality;Even though 4.0 factory requires significant investment, it will change traditional production relationships among suppliers, producers, and customers - as well as between human and machine – and lead to a reduction on development time and costs of production and operation, thus leading to greater efficiency.
Key Actions:
To achieve PARE objective 31, it is recommended to consider the best combination of 4.0 technologies, including automation and information, as applicable to various production rates and scales of equipment in aeronautics.
TELECOMMUNICATIONS
Telecommunications is a major innovation and growth sector in modern societies that become readily embedded in aeronautics technologies and the services they provide, including navigation and air traffic management (ATM), on-board monitoring and preventive maintenance, flight safety and security, etc. The expected growth of air traffic and consequently the need for increased air traffic capacity and safer and more efficient aircraft operations will require increased data sharing and larger telecommunications bandwidth protected from unintended or malicious interference. Taking this into account, by 2050, the telecommunications capacity needed for connected aircraft, navigation, monitoring and other services must be provided.
Key Findings:
The cluster of air traffic management (ATM), communication, navigation and surveillance (CNS), meteorological (MET) and aeronautical information (AIS) services are called air navigation services. Likewise, ATM services are divided into three different services: air traffic flow management (ATFM), airspace management (ASM) and air traffic services (ATS), which are composed in turn by air traffic control (ATC), flight information services (AIS) and alert services (AL);Telecommunications are the core of this network in such a way that they allow, for example, communications air to air, air to ground, ground to ground, as well as aircraft navigation or surveillance through the application of radio frequencies. Thereby, available communication and surveillance systems are essential for the correct functioning and effectiveness of ATC services as well as equipment based on radio waves is essential for the correct aircraft navigation; As a consequence of the growth of air traffic and, likewise, of the growth of the number of Navigational Aids (NAVAIDs), the need for a higher number of radio channels was identified due to the congestion of radio spectrum. This change in the frequency assignment has managed to increase the number of available channels in very high frequency (VHF) band of aeronautical communications, which has allowed the creation of new control sectors and has contributed to increasing the ATM capacity;The future scenario of communications relies on the use of the Aeronautical Mobile Satellite Service (AMSS) through the use of satellite communication (SATCOM) as well as the use of data link via VHF and high frequency (HF). Data link is a method for connecting one location to another in telecommunications, to transmit and receive digital information. However, in aeronautics data link is a generic term encompassing different types of data links systems and subnetworks;Satellite-based navigation will transform ATM because it will allow attaining the full implement of area navigation (RNAV). Besides, its precise time reference is expected to be used in surveillance and communications strategic applications. Therefore, within Global Navigation Satellite System (GNSS) network, the development and deployment of GALILEO system will be essential for the EU owing to GALILEO has been designed to fulfil all the required features for aviation users hence it can be widely used, unlike GPS which is hardly certifiable. In this manner, GALILEO will be more accurate and reliable than GPS and it will also inform users about possible system failures.
Key Actions:
To achieve PARE objective 32, it is recommended to assess the growth of capacity needs for navigation, systems monitoring and passenger services, and how the required bandwidth can provide free from unintended or malicious interference.
CYBERSECURITY
The increasing reliance on computer systems tied by telecommunications links (more interconnected systems) multiplies the entry points for disruptive and malicious intrusions, which can compromise major systems and lead to unlawful physical acts like hijack of aircraft, destruction of an aircraft in service or hostage-taking on board an aircraft or at airports. Considering this, the global aviation industry has many layers overseeing the safety of all the stakeholders involved, from aircraft manufacturers to the passenger boarding a flight. Aeronautics needs no less and perhaps more than many other sectors to implement security measures to safeguard civil aviation against these acts of unlawful interference. Thus, by 2050, threats in cybersecurity must be monitored and timely protection must be devised for all levels of aviation systems.
Key Findings:
Cybersecurity is about the prevention of and/or reaction to deliberate malicious acts undertaken via cyberspace (i.e. the domain of information flow and communication between computer systems and networks and includes physical as well as purely virtual elements) to either compromise the system directly or wherever systems play a key role. Airport and ATM cybersecurity is aimed at limiting the effects of such cyber-threats and the impact on airport organization and operations and the overall ATM network;One of the keys to fighting successfully against cyberattacks is to identify the potential cyber attackers, normally divided in 4 groups: state cyber-forces, who have a large number of resources at their disposal, state backing and are very highly skilled); cyber-criminals (who are interested in making money through fraud or from the sale of valuable information); terrorists (who are interested in obtaining and using sensitive information to launch a conventional attack); and hacktivists (who have a cause to fight for such as political or ideological motives);Cybersecurity has become a real issue for aviation, driven by different factors such as:
i) the increasing interconnectivity of ATM which means that the impact of an attack may extend across a growing number of interconnected systems;
ii) the increased reliance on integrated data which means a high potential for operational disruptions if connectivity is lost;
iii) the migration toward common and Commercial Off The Shelf (COTS) components, underpinned by industry-standard protocols such as Internet Protocol (IP), with published vulnerabilities which means that more people will have the technical background to launch attacks and more people will have access to core infrastructures through extended supply chains; and
iv) new methods of attack stemming from either criminal activities and/or state-sponsored actors, of increasing levels of sophistication;
Cyber-vulnerabilities have also been exposed at industry and government level, even in the largest aerospace programmes, like the F-35 Lightning II – Joint Strike Fighter Programme: a) industrial espionage has used small sub-contractors with modest security resources to access up the supply chain to main contractors; b) intergovernmental meetings among the representatives of participating nations have had uninvited, invisible “guests” from other nations;To ensure the identification of appropriate preventive security measures, the level of threat should be continually reviewed, and risk assessments carried out, taking into account international, national and regional situations and environments. In this manner, whenever a specific threat exists, selected and predetermined preventive security measures should be applied, commensurate with the associated risk assessment and the nature and severity of the threat.
Key Actions:
To accomplish PARE objective 33, it is recommended to consider the levels of cyber protection needed for the various aeronautical activities, and how to monitor and counter threats.
BIG DATA
The new generation of aircraft generates a lot of data from multiple sources: flight tracking data, airport operations data, meteorological data, airline information, market information, passenger information, aircraft information and air safety. Some of these data can be obtained through thousands of sensors and sophisticated digitalised systems that are installed on-board an aircraft. Using big data analytics to process efficiently the great amount of data from different sources can have several applications on aviation: optimising fuel consumption, crew deployment and flight operations, reducing cost, improving maintenance, forecasting weather and air safety, and enhancing passenger experience; that will become standard to support real-time decision making. By 2050, the implications in aeronautics of advances in big data, including the use of what is already available must be assessed.
Key Findings:
Currently, the aviation industry adopts on condition/preventive maintenance procedures due to its operational efficiency and it depends upon the failure mode calculations made after testing a part under circumstances. These conditions may fluctuate depending on the external factors/human errors which may result in the variation in the lifetime of components, in turn, reducing the operational efficiency of the aircraft;A study by the Federal Aviation Administration (FAA) states that during a year a jet engine generates data equivalent to 20 terabytes (TB). Most of this data is not used for any of the analytics purposes since this data is unstructured. Big data analytics can be used to predict the fault in the component by analysing data obtained from various sensors and account of the specific component attached to an aircraft or fleet in which it is present;A typical aircraft has millions of parts. The amount of data output is immense and is increasing rapidly with a new generation of aircraft. Legacy aircraft used to capture more than 125 flight parameters. The Airbus A320, for example, produces 20,000 data parameters but the latest A350 produces 400,000 and has a data output of approximately 250 gigabytes (GB) per flight. Boeing 787 captures more than 1000 flight parameters with some reports claiming half a terabyte of data per flight; Nowadays, data mining, which is a broad field of data science used to make predictions on future data based on patterns found in collected data, can be used to improve aviation safety by analysing all the data collected from the reports and searching for patterns and anomalies that indicate potential incidents and hazardous situations before they happen. However, this method presents several limitations, e.g. the requirement of some knowledge of what should be searched for and found and therefore, the necessity of a method that determines the relevant and important data. Besides, as the data included in reports is overly generalised, it is difficult to identify unknown patterns, and, in consequence, data mining usually discovers known trends.
Key Actions:
To achieve PARE objective 34, it is recommended that the expected benefits versus the required investment in using big data techniques in aeronautical activities for which relevant data already exists or has to be newly sourced are considered.
ARTIFICIAL INTELLIGENCE
Artificial intelligence (AI) has the advantage of systematic learning from large amounts of data (‘Artificial Imitation’) and therefore it can lead to the more efficient and reliable implementation of known tasks. However, it lacks any form of imagination when facing unforeseen or untrained situations, when it cannot replace human imagination, resourcefulness or true and real intelligence. Considering this, by 2050, the potential benefits and risks of the use of AI in aeronautics must be assessed.
Key Findings:
Some existing AI use cases in aviation include:
Flight management computer systems used to assist the pilot with information and for handling purposes;Computer systems behind the autopilot feature to steer the aircraft along a predefined trajectory without the need for pilot intervention;Computer systems supporting automated aircraft cabin pressurization to ensure the cabin environment is safe and comfortable for passengers; Analysis and prediction of passenger behaviour/demand;Seamless airport security processes, through facial recognition and biometrics, currently being used to perform customer identity verification and to match passengers to their luggage through kiosks;Provision of support to the optimization of revenue management, route network, fleet management, and pricing strategies;Self-flying planes and autonomous in-flight services e.g. meals, snacks, beverages, duty-free shopping and others made available during a flight for the convenience of the passenger;Autonomous airport processes, e.g. ground handling, loading, fueling, cleaning, and aircraft safety checks;AI assistants that answer customer inquiries and voice commands for domestic airline flight information and ticket availability through interactions using natural language; andAeroplane simulators which process the data taken from simulated flights. One example is aircraft warfare where computers can come up with the best success scenarios in these situations as these machines can create strategies based on the placement, size, speed and strength of the forces and counter forces;
Despite all the benefits and advantages of AI, there are still many problems to overcome before its widespread applications: (i) dependence on large datasets (that can still have limited training data and lead to the failure of the model) and lack of abstraction and generalisability; (ii) lack of interpretation of the obtained trained models and use of current AI approaches as a black box; and (iii) lack of robustness, being that most current AI systems can be easily fooled, which is a problem that affects almost all machine learning techniques.
Key Actions:
To achieve PARE objective 35, it is recommended that the situations in aeronautics in which the learning processes of AI can be efficient and safe are identified and those where there is limited training material or potential dependence on imagination are distinguished.
NEW MATERIALS AND STRUCTURES
The development of new materials and structures employing them is driven both by the pull of aviation needs and by the progress of technologies. The contribution of materials and structures to progress in aeronautics has been steady and gradual, e.g. a 10-30% weight saving replacing a metal wing or fuselage by a full composite one, and the prospect of structures having a fraction of their current weight is brought by nanotube developments. Considering this, by 2050, the implications on aircraft design, certification, operation and maintenance of new developmental and breakthrough materials and structures, including nanotube structures and microelectromechanical devices, must be assessed.
Key Findings:
Progress in materials has been a key to advances in aeronautics since its birth at least in two areas: (i) strong and light structures able to withstand flight and landing loads without demanding more than the essential lift and incurring excessive drag; (ii) high-temperature turbine blades of jet engines subject to rotational and aerodynamic loads combined with heat fluxes;Forty years ago aluminium dominated the aerospace industry - as much as 70% of an aircraft was made of it, from the fuselage to main engine components. Materials such as titanium, graphite, fibreglass or composites and alloys were also used, but only in very small quantities. Nowadays, a typical aeroplane is constructed as little as 20% pure aluminium and most of the non-critical structural material consists of even lighter-weight carbon fibre reinforced polymers (CFRPs) and honeycomb materials;While CFRPs represent the lion’s share of composite material in both cabin and functional components, and honeycomb materials provide effective and lightweight internal structural components, next-generation materials include ceramic-matrix composites (CMCs), which are emerging in practical use after decades of testing;For engine parts and critical components, there is a simultaneous push for lower weight, increase strength and higher temperature resistance, intending to reduce the cost of aerospace manufacture, improve fuel economy through efficiency and light-weighting. Considering that the melting point of current superalloys is around 1,850°C, to meet these temperature demands, heat-resistant super alloys (HRSAs), including titanium alloys, nickel alloys, and some non-metal composite materials such as ceramics, are now being brought into the material equation;The major drivers in current aerospace R&D are towards lighter construction materials and more efficient engines - the overall goal being to reduce fuel consumption and carbon emissions associated with air travel and air freight. The significant interest in nanotechnology for the aerospace industry is justified by the potential of nanomaterials and nanoengineering to help the industry achieve this environmental goal.
Key Actions:
To achieve PARE objective 36, it is recommended that the progress in advanced materials like composites, ceramics and special alloys and also the prospects of less mature developments like nanotube structures and other nanotechnologies is considered.
FOR MORE INFORMATION ABOUT THESE TOPICS, EXPLORE THE FULL CHAPTER